Genetic Engineering: Revolutionizing Agriculture with Pest-Resistant and Drought-Tolerant Crops
Published on: May 5, 2025
Genetic Engineering and Its Impact on Modern Agriculture
Genetic engineering has emerged as one of the most transformative technologies in the field of agriculture. By directly manipulating the genetic material of crops, scientists have been able to develop new varieties that are resistant to pests and diseases, tolerant to drought and other environmental stresses, and even enhanced with improved nutritional profiles. This revolution in plant breeding is not only increasing productivity, but also reducing the need for chemical inputs and helping to address some of the most pressing challenges facing global food production.
The Science Behind Genetic Engineering in Plants
At its core, genetic engineering involves the direct modification of an organism’s DNA using biotechnological techniques. In agriculture, this typically means introducing specific genes into the genome of a crop plant to confer desirable traits. This process can involve the transfer of genes from unrelated species, a technique that distinguishes it from traditional breeding methods which rely on the crossing of closely related plants.
One of the earliest and most widely used techniques is the use of Agrobacterium tumefaciens, a soil bacterium that naturally transfers DNA to plants. Scientists harness this capability to insert genes that code for beneficial traits. Other methods include the use of gene guns, which physically shoot DNA-coated particles into plant cells, and more recently, genome editing technologies like CRISPR-Cas9, which allow for precise changes to be made at specific locations in the plant genome.
Genetic engineering has enabled the development of crops with traits that would be difficult or impossible to achieve through conventional breeding. These include resistance to specific insect pests, tolerance to herbicides, enhanced nutritional content, and the ability to withstand abiotic stresses such as drought and salinity.
Pest-Resistant Crops: Reducing the Need for Pesticides
One of the most significant applications of genetic engineering in agriculture has been the creation of pest-resistant crops. The introduction of Bacillus thuringiensis (Bt) genes into crops such as cotton, maize, and soybean has led to varieties that produce their own insecticidal proteins, targeting specific pests while being harmless to humans and most beneficial insects.
Bt crops have had a profound impact on pest management strategies. In countries like the United States, India, and China, the adoption of Bt cotton has resulted in substantial reductions in the use of chemical insecticides. For example, studies have shown that Bt cotton in India reduced pesticide applications by up to 50%, leading to lower production costs, reduced environmental contamination, and improved health outcomes for farmers.
Moreover, pest-resistant genetically modified (GM) crops can contribute to sustainable agriculture by reducing the risk of pest outbreaks and the development of resistance to conventional pesticides. However, careful management and monitoring are required to prevent pests from evolving resistance to the Bt proteins, which could undermine the long-term effectiveness of these crops.
Drought-Tolerant Crops: Addressing Water Scarcity
Water scarcity is a growing concern in many parts of the world, threatening agricultural productivity and food security. Genetic engineering has enabled the development of crops that can maintain yields under conditions of limited water availability. Drought-tolerant GM crops achieve this through various mechanisms, such as improved root systems, enhanced water-use efficiency, and the ability to maintain cellular function during periods of water stress.
One notable example is the development of drought-tolerant maize varieties, which have been commercialized in the United States, Africa, and other regions. These varieties have demonstrated yield advantages over conventional maize under moderate drought conditions, offering farmers a valuable tool to cope with increasingly erratic weather patterns associated with climate change.
Beyond maize, research is ongoing to develop drought-tolerant varieties of other staple crops, including rice, wheat, and sorghum. The successful deployment of these crops could help stabilize food production in vulnerable regions and reduce the risk of crop failure due to drought.
Enhancing Crop Nutrition Through Genetic Engineering
Malnutrition remains a serious problem in many developing countries, where diets often lack essential vitamins and minerals. Genetic engineering offers a way to address this challenge by creating crops with improved nutritional content. This approach, known as biofortification, involves increasing the levels of specific nutrients in staple foods.
One of the most well-known examples of a biofortified GM crop is Golden Rice, which has been engineered to produce beta-carotene, a precursor of vitamin A. Vitamin A deficiency is a leading cause of preventable blindness and increases the risk of disease and death in children. Golden Rice has the potential to provide a significant portion of the daily vitamin A requirement for populations that rely heavily on rice as a staple food.
Other biofortified crops developed through genetic engineering include cassava with increased iron and zinc content, maize with enhanced levels of essential amino acids, and potatoes with higher vitamin C content. These innovations have the potential to improve public health outcomes and reduce the burden of micronutrient deficiencies in vulnerable populations.
Reducing Agriculture’s Environmental Footprint
Traditional agricultural practices often rely heavily on chemical fertilizers, pesticides, and irrigation, all of which can have negative environmental impacts. By enabling crops to resist pests and tolerate drought, genetic engineering can reduce the need for these inputs, leading to more sustainable farming systems.
Pest-resistant crops decrease the use of chemical insecticides, which not only benefits the environment but also protects the health of farm workers and communities. Drought-tolerant crops reduce the demand for irrigation, conserving water resources and lowering the energy required for pumping and distribution. In addition, some GM crops have been engineered for improved nitrogen use efficiency, reducing the need for synthetic fertilizers and minimizing nutrient runoff into waterways.
These benefits contribute to a smaller ecological footprint for agriculture, helping to preserve biodiversity, maintain soil health, and mitigate the impacts of climate change.
Challenges and Concerns Associated with Genetically Modified Crops
Despite the many benefits, the use of genetically modified crops remains a subject of debate and controversy. Concerns have been raised about the potential ecological risks, such as gene flow to wild relatives, the development of resistant pests and weeds, and unintended effects on non-target organisms.
There are also social and economic issues to consider, including the concentration of seed ownership among a few large biotechnology companies, the impact on smallholder farmers, and the need for transparent regulatory frameworks. Public perception and acceptance of GM crops vary widely across regions, with some countries embracing the technology and others imposing strict bans or labeling requirements.
Scientific consensus holds that currently approved GM crops are as safe to eat as their conventional counterparts, and that the environmental risks can be managed through appropriate stewardship and regulatory oversight. Ongoing research and monitoring are essential to ensure that the benefits of genetic engineering are realized while minimizing potential risks.
Regulation and Safety Assessment of Genetically Modified Crops
Before genetically modified crops are commercialized, they undergo rigorous safety assessments to evaluate potential risks to human health and the environment. Regulatory agencies such as the United States Department of Agriculture (USDA), the Environmental Protection Agency (EPA), and the Food and Drug Administration (FDA) in the US, as well as similar bodies in other countries, review data on the genetic modification, food safety, allergenicity, toxicity, and environmental impact.
These assessments typically involve laboratory and field studies, as well as reviews of the molecular characterization of the introduced genes and their expression in the plant. In addition, post-market monitoring is often required to detect any unforeseen effects after commercialization.
The regulatory process is designed to ensure that only GM crops that meet strict safety standards are approved for cultivation and consumption. International organizations such as the World Health Organization (WHO) and the Food and Agriculture Organization (FAO) provide guidance on risk assessment and harmonization of regulatory approaches.
Global Adoption of Genetically Modified Crops
Since the first commercial planting of GM crops in the mid-1990s, their adoption has grown rapidly in many parts of the world. According to the International Service for the Acquisition of Agri-biotech Applications (ISAAA), more than 190 million hectares of GM crops were cultivated globally in 2019, with the largest producers being the United States, Brazil, Argentina, Canada, and India.
The most widely grown GM crops include soybean, maize, cotton, and canola, with traits such as herbicide tolerance and insect resistance being the most common. In Africa and Asia, the adoption of GM crops is increasing, particularly as new varieties are developed to address local challenges such as drought, pests, and poor soil fertility.
However, the adoption of GM crops is not uniform. In Europe, for example, public opposition and regulatory restrictions have limited the cultivation of GM crops, although imports of GM animal feed are common. The global landscape is shaped by a complex interplay of scientific, regulatory, economic, and cultural factors.
Case Studies: Success Stories and Ongoing Research
- Bacillus thuringiensis (Bt) Cotton in India: Since its introduction in 2002, Bt cotton has been adopted by millions of Indian farmers. Studies have documented significant increases in yields, reductions in pesticide use, and improved incomes for smallholder farmers. However, challenges such as secondary pest outbreaks and resistance management remain important considerations.
- Drought-Tolerant Maize in Africa: The Water Efficient Maize for Africa (WEMA) project has developed drought-tolerant maize varieties using both conventional breeding and genetic engineering. Field trials in Kenya, Uganda, and other countries have shown yield benefits under water-limited conditions, contributing to food security for smallholder farmers.
- Golden Rice in Asia: After years of development and regulatory review, Golden Rice has been approved for cultivation in the Philippines and Bangladesh. It is expected to play a role in addressing vitamin A deficiency among populations that rely on rice as a staple food.
Ongoing research is focused on developing crops with resistance to emerging pests and diseases, improved tolerance to environmental stresses, and enhanced nutritional profiles. Advances in genome editing technologies such as CRISPR are enabling even more precise and efficient modifications, opening up new possibilities for crop improvement.
Socioeconomic Impacts of Genetically Modified Crops
The introduction of genetically modified crops has had significant socioeconomic impacts, particularly in developing countries. Increased crop yields and reduced input costs can lead to higher incomes for farmers, improved food security, and poverty reduction. For example, studies have estimated that the adoption of Bt cotton in India has contributed to a substantial increase in farm income and a reduction in rural poverty.
However, access to GM seeds and technologies can be a barrier for smallholder farmers, especially when intellectual property rights and high seed costs are involved. Efforts to develop public-sector GM crops and ensure affordable access are important for maximizing the benefits of genetic engineering for all farmers.
There is also a need to consider the broader social and cultural context, including traditional farming practices, consumer preferences, and the role of women in agriculture. Participatory approaches that involve farmers and local communities in the development and deployment of GM crops can help ensure that the technology meets the needs and priorities of diverse stakeholders.
Future Prospects for Genetic Engineering in Agriculture
The future of genetic engineering in agriculture is closely tied to advances in biotechnology and genomics. New tools such as CRISPR and other genome editing technologies are enabling scientists to make precise changes to crop genomes without introducing foreign DNA, potentially addressing some of the regulatory and public acceptance challenges associated with traditional GMOs.
Researchers are also exploring ways to engineer crops that can fix their own nitrogen, reducing the need for synthetic fertilizers, and to develop plants with enhanced resistance to multiple stresses, including heat, salinity, and pathogens. The integration of genetic engineering with digital agriculture, big data, and precision farming technologies holds promise for optimizing crop performance and resource use.
The ongoing challenge is to ensure that the benefits of genetic engineering are accessible to all, particularly smallholder farmers in developing countries, and that the technology is used in ways that are environmentally sustainable and socially equitable. Continued investment in research, capacity building, and international collaboration will be essential for realizing the full potential of genetic engineering to support global food security and sustainable agriculture.
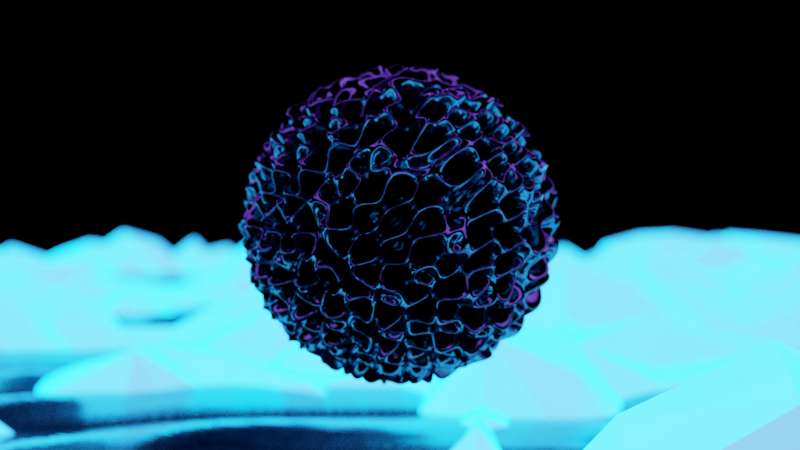